Self Therapeutic Nanomaterials
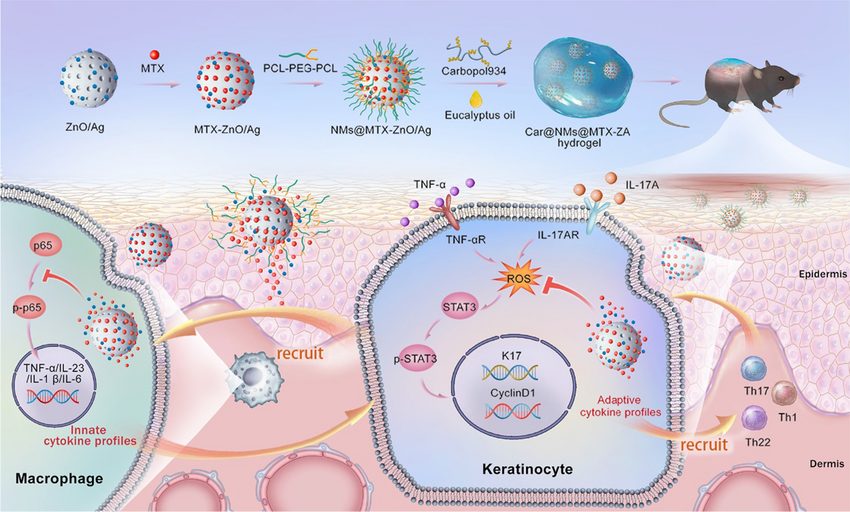
Self therapeutic nanomaterials (STNMs) utilize mechanisms ranging from selective surface adsorption to ion leaching in order to mediate cell–cell signaling, modulate biomolecular interactions, expose molecular manifestations of pathology, or catalyze substrate transformation
Over past decades, nanotechnology has contributed to the biomedical field in areas including detection, diagnosis, and drug delivery via opto-electronic properties or enhancement of biological effects. Though generally considered inert delivery vehicles, a plethora of past and present evidence demonstrates that nanomaterials also exude unique intrinsic biological activity based on composition, shape, and surface functionalization. These intrinsic biological activities, termed self-therapeutic properties, take several forms, including mediation of cell–cell interactions, modulation of interactions between biomolecules, catalytic amplification of biochemical reactions, and alteration of biological signal transduction events.
Moreover, study of biomolecule-nanomaterial interactions offers a promising avenue for uncovering the molecular mechanisms of biology and the evolution of disease. In this review, we observe the historical development, synthesis, and characterization of self-therapeutic nanomaterials. Next, we discuss nanomaterial interactions with biological systems, starting with administration and concluding with elimination.
Finally, we apply this materials perspective to advances in intrinsic nanotherapies across the biomedical field, from cancer therapy to treatment of microbial infections and tissue regeneration. We conclude with a description of self-therapeutic nanomaterials in clinical trials and share our perspective on the direction of the field in upcoming years.
Nanomatter is comprised of materials on the scale of 1 to 100 nm that exhibit properties not observed in similar bulk samples. Nanomaterial development has exploded within the biomedical community in recent decades in an attempt to harness these properties; as a result, biomedical nanomaterials have been shown to exhibit opto-electronic properties and selectively interact with biomacromolecules in ways not characteristic of either bulk materials or small-molecule therapeutics. While frequently utilized to accentuate the efficacy of other therapeutic modalities, many nanomaterials also display “self-therapeutic” properties based on chemical composition and nanoscale geometry. These self therapeutic nanomaterials (STNMs) utilize mechanisms ranging from selective surface adsorption to ion leaching in order to mediate cell–cell signaling, modulate biomolecular interactions, expose molecular manifestations of pathology, or catalyze substrate transformation. Herein, we review advances made in STNM technologies within the biomedical field. We highlight STNMs sequentially, offering notes on synthesis and characterization before overviewing the principles of interactions between STNMs and biological systems.
The history of nanoparticle (NP) therapy dates to the ancient world. Though macroscale gold was utilized medicinally for centuries prior, the elusive gold solution (“elixir of life”) was not synthesized until the 8th Century AD when Jabir ibn Hayyan produced gold tetrachloride in a solution of nitric and hydrochloric acid . For the next few centuries, potable gold was applied to myriad conditions and by 1890 was being utilized to treat diseases ranging from lupus to alcoholism. Since then, nanoscale gold has been noted to display properties of immune modulation and mediation of cellular interactions.
Current synthesis processes for nanoscale gold include chemical, biological, or physical methods, the most prevalent of which is the formation of gold nanoparticles (AuNPs) from HAuCl4, a reducing agent, and a stabilizing agent. Further methods exist for producing nanoscale gold of varying geometries, such as nanorods (AuNRs), nanoclusters (AuNCs), and nanoshells (AuNSs).
Like gold, silver has been touted for its therapeutic properties for centuries. Hippocrates was among the early proponents of its use, and it was most frequently applied to treating impairments of the central nervous system.
The antimicrobial properties of silver were harnessed to prevent food spoilage, wound infection, and even vertical transmission of venereal diseases. Currently, nanoscale silver is mainly utilized as an antimicrobial but has also been deployed in treatment of cancers, viral infections, and diseases of biomolecule aggregation.
Intriguingly, though silver nanoparticles (AgNPs) are potent antimicrobials, select microorganisms can synthesize AgNPs as a way to mitigate Ag ion toxicity. Silver nanostructures can also be formed via bottom-up chemical or biological methods or through top-down physical processes.
Though evidence suggests that the Egyptians had platinum in the 8th Century BC, it only became known to Europeans after its reemergence in the New World. Unlike other noble metals, platinum was rarely used medicinally until the discovery of cisplatin; since then platinum-based therapies have been a mainstay.
Platinum nanoparticles (PtNPs) are most commonly synthesized by the reduction of platinum salts with ethylene glycol or sodium borohydride as reducing agents, but PtNPs can also be synthesized through radiolytic reduction of platinum complexes or through biological methods.
Several types of metal oxide NPs exhibit intrinsic therapeutic properties. Magnetite (Fe3O4) iron oxide nanoparticles (IONPs) were recognized in the late 1980s for use as MRI contrast agents, and now IONPs composed of maghemite (γ-Fe2O3) and magnetite are commonly utilized in biomedical applications due to their low toxicity and superparamagnetic properties.
IONPs have been acknowledged as intrinsically therapeutic through induction of ferroptosis and conversion of hydrogen peroxide to hydroxyl radicals (termed the “Fenton reaction” after it was described by H.G.H. Fenton in 1894). Fenton-like chemistry has also been observed in other metal oxide NPs including tin (SnO2 NPs), manganese (MnO2 NPs), and molybdenum (MoO3-x NPs).
Zinc oxide NPs (ZnO NPs) do not display Fenton-like chemistry, but are antibacterial, treat diseases of bioaggregation, and mediate cellular pathways.
Finally, cuprous oxide nanoparticles (CuO NPs) display intrinsic catalytic activity in addition to inducing apoptosis in cancer cells.
Synthesis methods for metal oxide NPs include chemical, physical, and biological means, though chemical methods of coprecipitation, thermal decomposition, microemulsion, and Sol-gel techniques predominate.
Inorganic compounds such as calcium phosphates (CaPO4-family) also exhibit self-therapeutic properties. First mentioned around the turn of the 21st Century, NPs composed of calcium phosphate (CPNPs) were noted as non-viral gene delivery vectors before their self-therapeutic properties were elucidated.
Since then, CPNPs have been notable for antimicrobial properties along with immunogenicity and promotion of bone remodeling.
CPNPs of hydroxyapatite (Ca10(PO4)6(OH)2) and α/β-tricalcium phosphate (α/β-Ca3(PO4)2) have shown the greatest promise in bone remodeling by promoting osteoinduction and resorption.
CPNPs can be formed through chemical methods including precipitation, with process modifications allowing continuous synthesis or hybrid particle formation through co-precipitation.
Among the more recently developed STNMs are nanoclays: layered nanomaterials composed of hydrous silicates found in soil clay fractions.
Biomedical nanoclays vary in charge and include several compositions such as montmorillonite, kaolinite, laponite, halloysite, bentonite, hectorite, laponite, sepiolite, saponite, and vermiculite. For example, laponite was among the first to be recognized for its biomedical potential and displays a negative charge along its face and a positive charge along its edges.
Nanoclays have been utilized as bioadhesives and drug delivery systems, though most widespread use is found in tissue engineering. Methods of producing bioactive nanoclays vary and include 3D printing, self-assembly, and phase separation.
Though carbon-based materials have existed for millenia, the widespread use of carbon nanostructures in the biomedical field has been initiated within the last two decades. Carbon-based nanomaterials take several forms based on the atomic binding structure.
Nanodiamonds exhibit tetrahedral covalent bonding, while graphene nanosheets and quantum dots (GQDs) consist of single layers of covalently bonded carbon atoms.
Layers of carbon can also be formed into cylinders or shells to create bioactive nanotubes or nanospheres. Due to the variation in geometry and functionalization, intrinsic therapeutic properties of carbon-based nanomaterials have been applied throughout the biomedical field with emphasis on tissue engineering, prevention of biomolecular aggregation, and complementation of enzymatic activity.